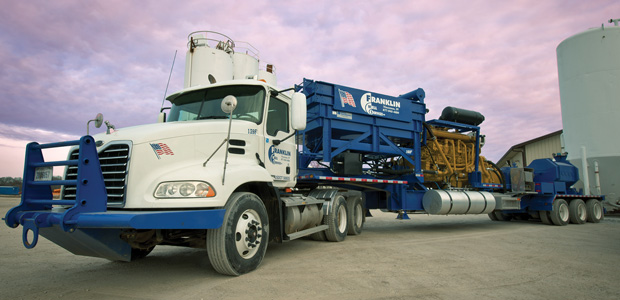
Frac Design
Resistivity Transform Indicates ‘Fracturability’
By Richard M. Bateman, Ahmed Alzahabi and Mohamed Y. Soliman
LUBBOCK, TX.–When working in unconventional reservoirs, the requirements of the formation evaluation analyst go beyond standard log and petrophysical analyses. In addition to assessing petrophysical and geochemical formation properties such as porosity, total organic content (TOC), mineral content and organic maturity, the analyst is often called on to predict geomechanical characteristics such as elastic properties.
From these studies, the ductility or brittleness of a given formation can be deduced from comprehensive logging suites. Numerous “fracturability” or “brittleness” indices have been proposed that are based either on mineral content (carbonate, silica, clay, etc.) or formation mechanical properties such as Young’s modulus and Poisson’s ratio. A common feature of all these indices is that they require multiple logging measurements as inputs to algorithms that compute “answer” parameters, which in turn, may or may not correlate with limited core analysis or results from monitored field trials.
The drawback of these indices, of course, is that not every well will have all the logging sensor measurements needed to compute the various algorithms. Many require both compressional and shear sonic slowness, bulk density, neutron porosity, photoelectric factor, T2 from magnetic resonance logs, and spectral gamma ray data on potassium, thorium and uranium concentrations. Such an abundance of petrophysical log data is seldom available for all wells in a field.
However, there is a petrophysical property of the formation that is universally available in all wells: resistivity. Resistivity data may be available from an induction or laterolog device, depending on the mud system used to drill the well. Formation resistivity is a parameter sensitive to the insulating properties of solid matrix materials and the conductive paths that are only available where there are interconnected pores with an ionic conductive fluid filler.
In conventional reservoirs, porous water-bearing intervals are conductive and nonporous intervals are resistive. In unconventional reservoirs, high kerogen-content organic shales are resistive and ductile (high clay-content) shales are conductive. Therefore, formation resistivity is an indicator of frac barriers where resistivity is low, as well as an indicator of harder, low-clay, high-TOC organic shales that are more brittle and easier to fracture.
‘Quick Look’ Indicator
Research suggests that a simple and straightforward transform of a deep resistivity curve is a useful surrogate for more complex indices that require multiple logging measurements. It has an important role to play in providing a “quick look,” one-stop fracturability indicator when only a resistivity curve is available at the time a hydraulic fracturing program is being designed.
Where full log suites are available and several mineralogical and/or geomechanical indices can be computed, the simpler resistivity index can be fine-tuned to a particular field in order to provide an improved (localized) version of the formula better suited to specific conditions at a given well site.
An interesting consequence of the Archie relationship is that the reciprocal square root of formation resistivity is linear with the formation’s bulk volume of water. In other words, the bulk volume of water, given by the product of porosity and water saturation, when squared, is proportional to the rock’s conductivity. This is the basis for the well-known Hingle plot used by log analysts, and indirectly, also is used in the Passey overlay as a means to determine TOC from sonic and resistivity logs.
With the Hingle method, log analysts use the reciprocal square root of deep resistivity (Rt) as a tool to help define the formation matrix material and identify water- and hydrocarbon-bearing zones from the data read from any pair of resistivity and porosity logs. It is usually abbreviated as RRRT. In highly resistive and hard formations, Rt will be high and RRRT will be low. In fact, an infinitely high-resistivity rock (essentially an insulator) would have a value of RRRT equal to zero. At the other end of the spectrum, a low-resistivity (conductive) ductile shale might have a resistivity of 1 ohm meters squared per meter (ohm-m) and an RRRT equal to 1.
Accordingly, RRRT neatly ranges from 0 in very hard (brittle) rocks to 1 in soft (ductile) formations. If a resistivity fracturability index (RFI) is defined so that it has a value of 0 in the least fracturable zones and a value of 1 in the most fracturable zones, than all that is needed is to compute the required index from the deep resistivity log. This algorithm may be generalized as RFI = A + B × RRRT.
Application Examples
For demonstration purposes, Figures 1-3 show how the resistivity index compares with more complex geomechanical and mineralogical indices that require log inputs of neutron, density and array sonic measurements. All three indices were computed using data from the same well.
In Figure 1, RFI is displayed over a log section of 600 feet. The better fracturing candidates are shown by “spikes,” with the best candidates showing values above 0.5. For comparison, Figure 2 shows a fracturability index based on geomechanical data, while Figure 3 is based on a mineralogical index. It is noticeable that the three indices are substantially in agreement.
The RFI has the potential to be tailored to imitate potentially any of these other indices on a local basis by the simple expedient of choosing an offset (A) and a scaling factor (B) to create, for example, a pseudo mineralogical index for use in the Eagle Ford or other shale play. The values of A and B can be chosen to render the rescaled RFI closest to the index to be mimicked.
This procedure can be followed not only for a particular play, but also for a given field within a play to account for variations in rock properties with depth or laterally from one area to another. In this way, a family of resistivity-based RFIs could be accumulated to fill in the gaps caused by a lack of full logging suites in all wells in a field. An experienced petrophysicist will be able to imitate both the mineralogical and geomechanical indices.
This simple algebraic transformation of universally available deep resistivity data can offer a surrogate index to optimize completion designs by identifying the most promising depths or parts of horizontal lateral intervals for hydraulic fracturing in shale and tight gas reservoirs.
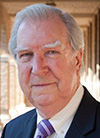
Richard M. Bateman is an associate professor in the Bob L. Herd Department of Petroleum Engineering at Texas Tech University. With research interests focused on petrophysics and well log interpretation, Bateman holds a B.A. and an M.A. in natural science and physics from Oxford University.
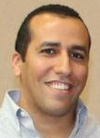
Ahmed Alzahabi is a research fellow and Ph.D. candidate in petroleum engineering at Texas Tech University. He is a member of the Global Leadership Certificate Program of Texas Tech, a fellow of the Society of Petrophysicists & Log Analysts, and technical editor of the Society of Petroleum Engineers’ Reservoir Evaluation & Engineering journal. Alzahabi holds an M.S. in petroleum engineering from Cairo University and a B.S. in petroleum engineering from Al-Azhar University.
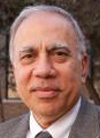
Mohamed Y. Soliman is the William C. Miller professor and chair of the petroleum engineering department at the University of Houston. He previously chaired the petroleum engineering department at Texas Tech, and worked for Halliburton for more than 30 years in technical and managerial positions. Soliman is a distinguished member of the Society of Petroleum Engineers, a fellow of the National Academy of Innovation, and a professional engineer in Texas. He holds a B.S. in petroleum engineering from Cairo University, and an M.S. and a Ph.D. in petroleum engineering from Stanford University.
For other great articles about exploration, drilling, completions and production, subscribe to The American Oil & Gas Reporter and bookmark www.aogr.com.