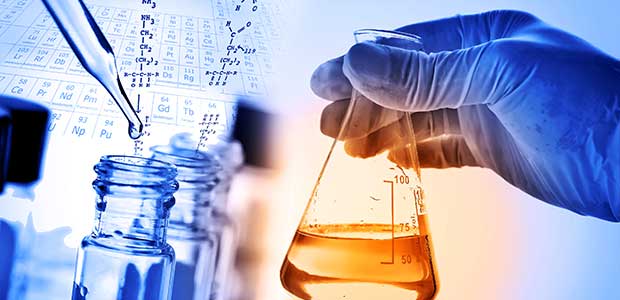
Proactive Chemistry
Chemical Treatments Mitigate Costly Problems When Restarting Shut-In Wells
By Rick McCurdy
OKLAHOMA CITY–The oil and gas industry has learned many lessons over the years, but one of the most frustrating and painful is a recurring lesson: The prices for the industry’s commodity products are always in a process of changing, and they can fluctuate wildly over time. The current pricing environment continues to improve, but it is important to remember that there have been many times in the past when market prices for both oil and natural gas have fallen to levels where it cost more to bring the products out of the ground and prepare them for sale than the actual value gained by selling them.
The second quarter of 2020 was unlike anything the industry had experienced. The scale of well shut-ins in early spring and the circumstances behind it may have been unprecedented, but the practice of shutting in wells during periods of sub-breakeven prices was not. In the conventional oil and gas production space, operators long ago adapted to uneconomical price scenarios by simply shutting in oil or gas wells and waiting for prices to improve.
In trying to restart extended shut-ins in the past, operators have learned another costly lesson: Conventional wells that were shut in without proper chemical conditioning routinely suffered damage, primarily from both acid gas corrosion and microbiologically influenced corrosion (MIC). In the unconventional world, operators now are learning that same lesson still applies as they start bringing horizontal and conventional vertical wells back on line.
Ounce Of Prevention
Through its past experiences, the industry learned that the old adage “an ounce of prevention is worth a pound of cure” was indeed true in conventional vertical wells, and most operators began employing chemical conditioning treatments to protect wellbores and equipment whenever shut-ins had to be endured for an extended time. Unfortunately, the knowledge gained the hard way in conventional wells has not fully migrated to unconventional production operations, partially because of the relatively few instances where unconventional wells have had to be shut in for lengthy periods.
Proactive application of chemical treatments prior to shutting in an unconventional oil well can eliminate potentially costly problems when the time comes to restart production, including the deposition of paraffin and corrosion caused by the formation of carbon dioxide and hydrogen sulfide or bacterial growth. Shown here is damage to tubing caused by downhole bacteria.
However, the sudden collapse in oil prices in early March, followed by lockdown measures in response to COVID-19, resulted in many operators having to simply shut down wells for an unknown period and wait for better days and improved prices. While gas wells and conventional oil wells certainly were affected, most of the shut-in production volume came from unconventional oil wells. What might companies expect as they work to restore production in these horizontal wells?
The majority of unconventional oil wells produce some associated gas. Like their conventional counterparts, unconventional formations yield natural gas that almost always contains some fraction of carbon dioxide, and occasionally also may contain hydrogen sulfide, a potentially poisonous gas.
Henry’s Law informs us that when a gas and a liquid are together and pressure is applied, part of the gas diffuses or dissolves into the liquid. As such, both CO2 and H2S (if present) can be present in an unconventional well as weak acids in the brine. Both CO2 and H2S have been shown to corrode downhole metals, including those used in pump systems, tubulars and rods.
Operators protect their downhole tubing (and rods if the well is on artificial lift with an insert or tubing pump) from these corrosive forces with either constant injection or periodic batch application of corrosion inhibitor. Corrosion inhibitors lay down a light film on the metal surface to prevent corrosive interaction with the produced water’s weak acids. Depending on the type of inhibitor being applied, the films range from very weak to somewhat tenacious, but none are intended as a permanent solution.
For conventional wells scheduled for shut-in, the industry has developed a standard practice of circulating an amount of film-forming inhibitor and also placing a water-soluble inhibitor in the flush fluid to protect subsurface assets during the shut-in period. As noted, it is highly unlikely that this practice was applied to many unconventional wells shut in last spring.
During shut-in, any previously applied corrosion inhibiting film will begin to wane and corrosion from weak acids potentially will begin. The likelihood and severity of this attack depends on four key factors:
- Whether the metal surface is in contact with produced brine;
- The temperature of the brine at the point of contact and the pressure in the well;
- The types and levels of acid gases present in the brine; and
- The duration of the shut-in.
Greatest Risk
The corrosion cell requires a conductive solution (brine) for the electron transfer necessary for an electro-chemical reaction. Therefore, tubulars or rods that are not in contact with brine face little corrosion risk. Likewise, where gravimetric partitioning in the annulus and tubing have allowed oil and water to separate, tubulars and rods in contact with the oil will see little risk. Depending on a particular well’s oil/water cut and reservoir pressure, the greatest corrosion risk will be in the lower part of the production string, where reservoir temperature and wellbore pressure are at or near maximum. Such pressure drives more acid gas into solution in the brine. Corrosion rates increase with temperature, making the lateral and lower part of the well the highest risk segments for corrosion potential.
While weak acid concentration, temperature and pressure will determine the actual rate of any corrosion, the overall potential for downhole damage to rods and tubulars depends on the length of the shut-in.
As the well returns to production, steps should be taken to re-establish a corrosion film on the downhole equipment. If the well had been treated with periodic batch treatments with a corrosion inhibitor prior to shutting in, batch treatment should resume as quickly as possible, with the first treatment containing an abnormally large volume of corrosion inhibitor. If the well was shut in two-four weeks, the operator should consider two or three times the normal corrosion inhibitor application. If shut in longer than three weeks, the first treatment should be sized with four-five times the normal inhibitor volume. A preflush with a suitable surfactant should be placed in front of the inhibitor pill to wash off any possible corrosion byproducts on the tubing or rods.
If the well was treated continuously with a corrosion inhibitor down the tubing/casing annulus without the aid of a capillary injection line, there likely will be some fluid that may impair the corrosion inhibitor’s reach. To shorten the time needed to get inhibitor down hole and back to surface through the tubing, a mixture of 10 gallons of corrosion inhibitor and 20 barrels of clean lease brine can be pumped down the tubing/casing annulus.
Bacterial Corrosion
Bacteria have been found in oil and gas operations and studied for decades. Many different species such as acid-producing, iron-oxidizing, iron-reducing and sulfate-reducing bacteria have been known to contribute to metal corrosion in oil and gas wells. Some contribute to corrosion by secreting weak acids, and some, such as sulfate reducers, utilize the sulfate ion for respiration, eventually releasing what remains as H2S into the produced brine. Some bacteria have been found to be naturally occurring in a reservoir, while others have been introduced through drilling, completion or remedial workovers.
Bacteria are found in oil and gas production in two basic states, either planktonic (free floating) or sessile (attached). Although planktonic bacteria make some contribution to corrosion, the greatest degree of corrosive attack occurs when the bacteria become sessile and attach to the metal surface. During normal operations, the velocity of the produced fluid moving through the lateral and up the tubing string limits bacterial corrosion. While some bacteria have a stronger affinity for attachment (becoming sessile), all benefit from a stagnant fluid.
In addition, most operators monitor bacteria levels in production operations and apply an Environmental Protection Agency-registered biocide to sanitize the produced well, surface vessel or tankage when bacteria numbers reach a certain level.
Past shut-ins of conventional production taught the industry about the corrosion potential of bacteria present in wellbore brines. To offset or limit this corrosion, fluids used to circulate a corrosion inhibitor prior to the anticipated shut-in have been treated with a biocide compatible with the corrosion inhibitor. These usually were based on quaternary ammonium compounds (quats), or blends of quats with glutaraldehyde. Including these chemicals in the circulated fluid prevents or severely limits the growth of bacteria in the wellbore during the shut-in period.
Again, given the circumstances at the time, it is unlikely that shut-in unconventional oil wells were treated properly with a biocide pill. If bacteria have been known to be a problem in these wells or their surface equipment and tankage in the past, it is likely that bacteria will grow and become more sessile in the wellbore fluids. To limit damage to downhole tubulars and the integrity of surface separation equipment and tankage, wells returning to production should be treated down hole with a biocide pill of an EPA-registered biocide mixed with clean lease brine (assuming the brine is bacteria free) or 2% potassium chloride. This treatment can be in conjunction with surfactant/corrosion inhibitor treatment.
A biocide known to be compatible and harmless to the corrosion inhibitor film should be selected and applied at a rate of 500-1,000 parts per million. Post-treatment, the well brine should be tested for bacteria concentration to determine if a second sanitation is necessary.
Paraffin Waxes
The crude oils produced from unconventional wells often contain some percentage of paraffin waxes, organic compounds typically composed of 18-70 linear or branched carbon molecules. These wax molecules are almost always in solution in the produced crude under reservoir temperatures. The produced oil cools as it moves from the perforations and up the hole, eventually reaching the cloud point or wax appearance temperature. The wax appearance temperature is a function of the makeup of the wax molecules in the crude and the overall concentration or percentage of wax molecules.
At this temperature, the wax molecules begin to form crystals and platelets, and come out of solution. Wax appearance temperature often varies considerably across different unconventional oil formations, but it is generally common to see wax forming somewhere in the tubing string before the oil reaches the surface. Where exactly wax platelets begin to form and attach to the tubing’s internal diameter and the surface of the rods is a function of the wax concentration and overall molecular makeup, formation temperature and production rate.
A well producing a high rate of fluid often can carry fluid from the formation to surface before a substantial amount of formation temperature is lost, thereby limiting the amount of wax that may form in the tubing, wellhead or surface flowlines. Conversely, a well producing a small volume of fluid will see that production lose reservoir temperature much quicker and likely experience wax coming out of solution thousands of feet down hole.
When an unconventional oil well is shut in, the fluid trapped in the tubing (assuming it is not a flowing well) will begin to gravitationally separate. Brine will fall to the bottom of the tubing string and oil will remain on top. If a well with a 70-to-30 oil-to-water ratio and 10,000 feet of tubing is shut in, it will have 7,000 feet of crude in the tubing sitting on top of 3,000 feet of brine. Because the well is not producing to surface, formation temperature is not moving up hole with the produced fluid, and the oil and brine quickly assimilate to the surrounding rock temperature at their respective depths.
A well that previously experienced downhole wax formation beginning at 3,000 feet and upward now may deposit wax much lower. Additionally, a lengthy shut-in also may result in a larger volume of downhole wax.
Limited Options
Chemically, operators have few options to address existing paraffin when returning a shut-in well to production. Some of the precipitated wax is likely to enter the surface equipment and tankage as the well begins producing fluid and can be treated there using chemical or thermal means. If the wax precipitated down hole is sufficiently severe–making it difficult to operate the well–it may be necessary to thermally treat down the annulus with either hot oil or hot water to allow some of the wax to melt and move with the produced fluids.
Previous work has demonstrated that heat applied through fluids at surface are limited in how far that heat extends down hole. Based on this, thermal treatment may not sufficiently remove wax that formed deep in the tubing during shut-in.
If this seems to be the case, batching a volume of a paraffin solvent/dispersant down the tubing/casing annulus, where it will be produced back up the tubing, can help. Doing this while placing the well on total produced fluid circulation has proven very effective with wax removal using a solvent/dispersant. The well should be circulated to allow for at least two passes of the solvent/dispersant through the tubing string. Determining the effectiveness of wax removal after shut-in can be done through monitoring amperage on prime mover motors, flowline pressure and “grind outs” of oil from surface vessels and tanks.
Unfortunately, the question is likely not whether the industry will face another time where unconventional wells need to be shut in, but when. Operators should establish a standard practice for protecting their valuable assets from corrosion and paraffin by proactively applying chemistry prior to shutting those wells in. After all, an ounce of prevention . . .
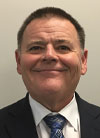
RICK MCCURDY is chief technology officer at maxSWD LLC, a chemical supplier helping midstream water operators optimize flow assurance and injection in saltwater disposal wells. In addition, he is the principal consultant for RMc3 Consulting LLC, providing chemistry, conservation (water reuse) and corrosion consulting services. McCurdy previously served as a subject matter expert in chemistry, corrosion, produced water treatment and reuse, and alternative water sourcing for hydraulic fracturing at Chesapeake Energy Corp. He was a primary developer of Chesapeake’s initiative championing the beneficial use of produced water, AquaRenew®, and its GreenFrac® environmentally-friendly additives program. McCurdy has served as a technical expert during U.S. Environmental Protection Agency workshops on hydraulic fracturing’s potential impacts on water resources, and has presented to the National Academy of Sciences, Engineering & Medicine; the Government Accountability Office; and the U.S. Department of Energy regarding water use in the energy sector. He holds an A.A.S. in petroleum technology.
For other great articles about exploration, drilling, completions and production, subscribe to The American Oil & Gas Reporter and bookmark www.aogr.com.